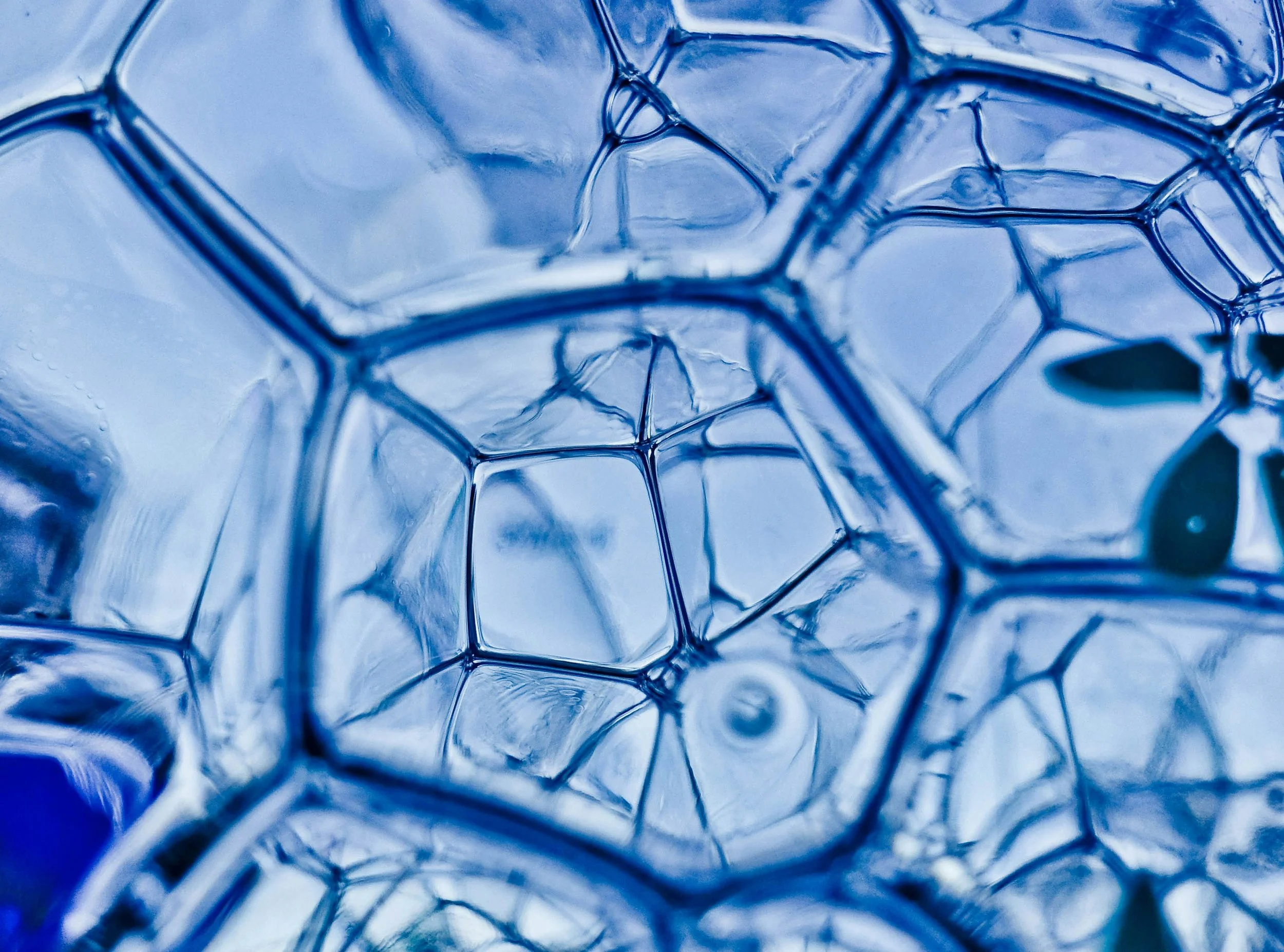
Mitochondria + Cellular Energy
Mitochondria are the powerhouses of our cells, driving every process in our body that requires energy. These microscopic organelles produce the ATP (adenosine triphosphate) that fuels nearly all cellular functions, from muscle contraction to brain activity. As the central hub for energy production, mitochondria are not only vital for our physical stamina but also for our metabolic health, longevity, and resilience to stress. In this section, we’ll explore the science of mitochondrial biogenesis, how oxidative stress impacts mitochondrial function, and the crucial connection between mitochondrial health and overall well-being.
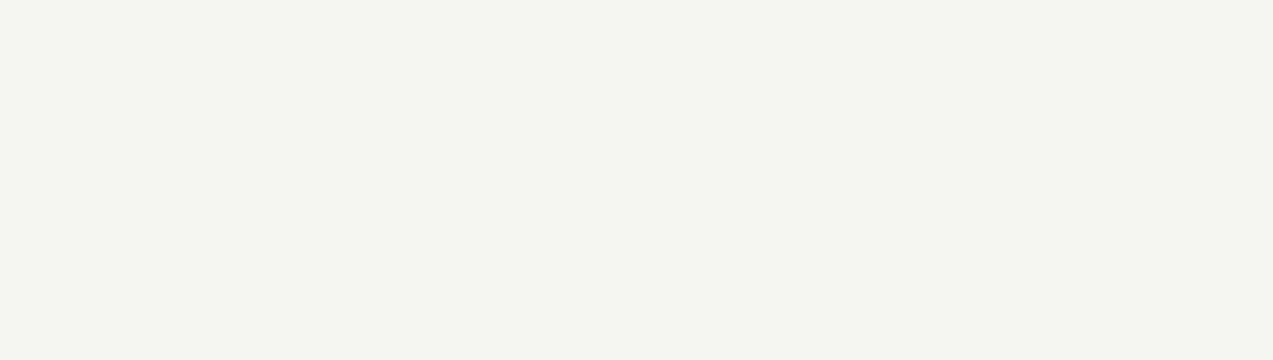
-
Mitochondria—the cell’s powerhouse—are central to energy production, cellular signaling, and redox balance. When mitochondria malfunction, it creates a ripple effect contributing to chronic diseases like metabolic syndrome, cardiovascular disease, neurodegeneration, and even cancer. Understanding the role of mitochondrial health offers a new lens through which to approach disease prevention and optimization.
1. Mitochondria and Energy Imbalance
Mitochondria generate ATP via oxidative phosphorylation (OXPHOS).
Dysfunction leads to impaired ATP production, forcing cells to rely on glycolysis, a less efficient and inflammation-promoting pathway.
Wallace (2005) emphasized mitochondrial dysfunction as a unifying factor in age-related and chronic diseases.
2. Mitochondria and Oxidative Stress
Malfunctioning mitochondria produce excess reactive oxygen species (ROS).
Elevated ROS damages lipids, proteins, and DNA, triggering inflammation and metabolic dysregulation.
Green et al. (2011) linked mitochondrial-derived ROS to insulin resistance and endothelial dysfunction.
3. Mitochondria’s Role in Inflammation
Damaged mitochondria release mitochondrial DNA (mtDNA) and other danger-associated molecular patterns (DAMPs), activating inflammatory pathways such as NLRP3 inflammasome.
West & Shadel (2017) detailed how mitochondrial DAMPs contribute to systemic inflammation and chronic disease.
Mitochondrial dysfunction sits at the crossroads of modern chronic disease. By supporting mitochondrial health, individuals can address root causes of metabolic, cardiovascular, and neurological conditions, building a foundation for lasting vitality.
References:
Wallace, D. C. (2005). A mitochondrial paradigm of metabolic and degenerative diseases, aging, and cancer: A dawn for evolutionary medicine. Annual Review of Genetics, 39, 359–407. DOI link
Green, K., et al. (2011). Mitochondrial reactive oxygen species mediate vascular endothelial dysfunction in insulin resistance. Arteriosclerosis, Thrombosis, and Vascular Biology, 31(2), 508–514. DOI link
West, A. P., & Shadel, G. S. (2017). Mitochondrial DNA in innate immune responses and inflammatory pathology. Nature Reviews Immunology, 17(6), 363–375. DOI link
-
Mitochondria are not just energy factories—they are sentinels of redox balance and immune regulation. Through the generation of reactive oxygen species (ROS) and control of inflammatory signaling pathways, mitochondria profoundly influence systemic inflammation and oxidative stress. When balanced, they foster cellular health; when dysregulated, they accelerate chronic disease.
1. Mitochondrial ROS: Friend and Foe
At low levels, mitochondrial ROS (mtROS) serve as signaling molecules that regulate autophagy, immunity, and cell survival.
At high levels, mtROS drive oxidative damage to DNA, proteins, and lipids, promoting disease progression.
Turrens (2003) described how mitochondria act as both a major source and target of ROS.
2. Mitochondria as Inflammatory Gatekeepers
Mitochondrial dysfunction leads to the release of mtDNA and cardiolipin, activating the NLRP3 inflammasome.
Nakahira et al. (2011) demonstrated that mitochondrial damage triggers inflammation via inflammasome activation in macrophages.
3. Antioxidant Systems within the Mitochondria
Mitochondria possess robust antioxidant defenses, including manganese superoxide dismutase (MnSOD) and glutathione.
Declining antioxidant capacity with age or poor diet exacerbates oxidative stress.
Mitochondria are at the heart of cellular redox and immune balance. Optimizing mitochondrial health provides a critical lever for reducing systemic inflammation and mitigating chronic disease.
References:
Turrens, J. F. (2003). Mitochondrial formation of reactive oxygen species. The Journal of Physiology, 552(2), 335–344. DOI link
Nakahira, K., et al. (2011). Autophagy proteins regulate innate immune responses by inhibiting the release of mitochondrial DNA mediated by the NALP3 inflammasome. Nature Immunology, 12(3), 222–230. DOI link
-
Mitochondrial biogenesis—the process of generating new mitochondria—is essential for optimal energy metabolism and cellular resilience. Emerging research highlights the synergistic role of key nutrients, dietary patterns, and lifestyle strategies in stimulating mitochondrial growth, function, and longevity.
1. Nutrients That Fuel Mitochondrial Health
Coenzyme Q10 (CoQ10): Supports electron transport chain efficiency and protects against oxidative damage.
Magnesium: Cofactor for ATP synthesis and mitochondrial enzyme activity.
PQQ (Pyrroloquinoline quinone): Promotes mitochondrial biogenesis via activation of PGC-1α, a master regulator of mitochondrial formation.
Rogers et al. (2014) outlined how PQQ supplementation enhances mitochondrial biogenesis in rodent models.
2. Mitochondrial Biogenesis and Fasting
Caloric restriction and intermittent fasting stimulate mitochondrial growth through AMPK and SIRT1 activation.
Civitarese et al. (2007) showed that calorie restriction upregulates PGC-1α and enhances mitochondrial function in humans.
3. Exercise-Induced Mitochondrial Adaptation
Both endurance and resistance training increase mitochondrial density and function.
Little et al. (2011) found that high-intensity interval training (HIIT) significantly upregulated mitochondrial biogenesis markers.
Mitochondrial biogenesis is a key strategy to enhance energy production, reduce oxidative stress, and support metabolic flexibility. Nutrition, movement, and fasting can powerfully stimulate this process.
References:
Rogers, G. B., et al. (2014). Pyrroloquinoline quinone stimulates mitochondrial biogenesis via activation of PGC-1α. Journal of Nutritional Biochemistry, 25(3), 237–244. DOI link
Civitarese, A. E., et al. (2007). Calorie restriction increases muscle mitochondrial biogenesis in healthy humans. PLoS Medicine, 4(3), e76. DOI link
Little, J. P., et al. (2011). A practical model of low-volume high-intensity interval training induces mitochondrial biogenesis in human skeletal muscle: potential mechanisms. Journal of Physiology, 588(6), 1011–1022. DOI link
-
Insulin resistance lies at the core of metabolic syndrome, type 2 diabetes, and cardiovascular disease. Emerging research highlights mitochondrial dysfunction as a key driver of insulin resistance, impairing glucose metabolism and promoting systemic inflammation.
1. The Mitochondria-Glucose Connection
Healthy mitochondria regulate glucose oxidation and fatty acid metabolism, preventing lipid accumulation.
Dysfunctional mitochondria reduce oxidative capacity, leading to ectopic lipid storage and disrupted insulin signaling.
Kelley et al. (2002) demonstrated that insulin-resistant individuals have reduced mitochondrial oxidative phosphorylation capacity.
2. ROS, Inflammation, and Insulin Resistance
Elevated mitochondrial ROS production exacerbates oxidative stress, triggering inflammatory cascades that impair insulin sensitivity.
Hoehn et al. (2009) showed that mitochondrial superoxide promotes insulin resistance in muscle cells.
3. Mitochondrial Adaptation via Nutritional and Lifestyle Strategies
Interventions such as exercise, caloric restriction, and nutrient-dense diets improve mitochondrial efficiency and insulin sensitivity.
Befroy et al. (2007) found that mitochondrial dysfunction in insulin-resistant patients could be reversed with lifestyle modifications.
Mitochondrial dysfunction is a key contributor to insulin resistance and metabolic disease. By restoring mitochondrial health, we can improve glucose regulation and reduce the risk of chronic illness.
References:
Kelley, D. E., et al. (2002). Dysfunction of mitochondria in human skeletal muscle in type 2 diabetes. Diabetes, 51(10), 2944–2950. DOI link
Hoehn, K. L., et al. (2009). Mitochondrial reactive oxygen species mediate adipocyte differentiation and insulin resistance. Nature, 453(7196), 1112–1116. DOI link
Befroy, D. E., et al. (2007). Impaired mitochondrial substrate oxidation in muscle of insulin-resistant offspring of type 2 diabetic patients. Diabetes, 56(5), 1376–1381. DOI link
-
Mitochondrial health is foundational to brain function. Neurodegenerative diseases like Alzheimer’s and Parkinson’s share common features of mitochondrial dysfunction, oxidative stress, and impaired energy metabolism, which precede clinical symptoms and drive disease progression.
1. Energy Deficits in the Brain
Neurons rely heavily on mitochondrial ATP for neurotransmission, synaptic plasticity, and neuroprotection.
Mattson et al. (2008) emphasized that bioenergetic failure due to mitochondrial decline is an early hallmark of cognitive decline.
2. Mitochondrial ROS and Neuroinflammation
Mitochondria-derived ROS activate microglia and astrocytes, promoting neuroinflammation and neuronal damage.
Lin & Beal (2006) outlined how mitochondrial dysfunction drives oxidative stress and chronic neuroinflammation in neurodegenerative disorders.
3. Mitochondrial DNA Damage
Accumulation of mtDNA mutations impairs electron transport chain function, further compromising neuronal energy balance.
Swerdlow (2018) explored how mtDNA mutations contribute to the mitochondrial cascade hypothesis of Alzheimer’s disease.
Mitochondrial dysfunction is a critical mechanism behind neurodegeneration. Strengthening mitochondrial resilience through nutrition, fasting, and exercise may protect brain health and slow cognitive decline.
References:
Mattson, M. P., et al. (2008). Energy intake and exercise as determinants of brain health and vulnerability to injury and disease. Cell Metabolism, 7(1), 7–20. DOI link
Lin, M. T., & Beal, M. F. (2006). Mitochondrial dysfunction and oxidative stress in neurodegenerative diseases. Nature, 443(7113), 787–795. DOI link
Swerdlow, R. H. (2018). Mitochondria and mitochondrial cascades in Alzheimer’s disease. Journal of Alzheimer’s Disease, 62(3), 1403–1416. DOI link
-
β-Hydroxybutyrate (BHB), a key ketone body produced during fasting or ketogenic diets, is more than an alternative fuel. BHB acts as a signaling molecule that modulates inflammation, enhances mitochondrial resilience, and protects against oxidative damage, making it a powerful ally for metabolic and mitochondrial health.
1. BHB as a Mitochondrial Fuel
BHB is metabolized efficiently within mitochondria, increasing ATP production while producing fewer reactive oxygen species compared to glucose.
Shimazu et al. (2013) demonstrated that BHB reduces oxidative stress by inhibiting class I histone deacetylases (HDACs), promoting antioxidant gene expression.
2. Anti-Inflammatory Signaling via BHB
BHB inhibits the NLRP3 inflammasome, reducing mitochondrial-induced inflammation.
Youm et al. (2015) found that BHB suppresses NLRP3 inflammasome activation, protecting cells from inflammation-induced damage.
3. Mitochondrial Biogenesis and Longevity Effects
BHB enhances PGC-1α activity and promotes mitochondrial biogenesis.
BHB signaling is linked to improved longevity markers and reduced cellular senescence.
BHB serves as a mitochondrial protectant, boosting ATP production while reducing oxidative and inflammatory stress. Harnessing ketone metabolism is a potent strategy to fortify mitochondrial health.
References:
Shimazu, T., et al. (2013). Suppression of oxidative stress by β-hydroxybutyrate, an endogenous histone deacetylase inhibitor. Science, 339(6116), 211–214. DOI link
Youm, Y. H., et al. (2015). The ketone metabolite β-hydroxybutyrate blocks NLRP3 inflammasome–mediated inflammatory disease. Nature Medicine, 21(3), 263–269. DOI link
-
Mitochondria are central to athletic performance and recovery. They fuel skeletal muscles during endurance and high-intensity exercise while orchestrating cellular repair and adaptation post-exercise. Optimizing mitochondrial function can significantly enhance athletic resilience and reduce recovery times.
1. Mitochondria and Aerobic Capacity
Mitochondria are responsible for aerobic ATP production, enabling sustained muscle contractions during prolonged exercise.
Holloszy (1967) was among the first to demonstrate that endurance training increases mitochondrial density in skeletal muscle.
2. Mitochondria and Recovery Processes
Mitochondrial biogenesis and mitophagy (removal of damaged mitochondria) are activated during exercise recovery.
Granata et al. (2018) reviewed how mitochondrial turnover is essential for muscle repair and adaptation.
3. ROS and Muscle Fatigue
Excessive mitochondrial ROS production during exercise contributes to muscle fatigue and delayed recovery.
Targeting ROS through antioxidant strategies can modulate recovery dynamics.
Mitochondria are crucial to exercise capacity and post-exercise recovery. Enhancing mitochondrial resilience improves athletic output and reduces the risk of overtraining.
References:
Holloszy, J. O. (1967). Biochemical adaptations in muscle: effects of exercise on mitochondrial oxygen uptake and respiratory enzyme activity in skeletal muscle. The Journal of Biological Chemistry, 242(9), 2278–2282. Link
Granata, C., et al. (2018). Mitochondrial adaptations to endurance training and the role of exercise-induced mitophagy in skeletal muscle remodeling. Free Radical Biology and Medicine, 122, 67–76. DOI link
-
Magnesium is an essential mineral required for over 300 enzymatic reactions, many of which are critical for mitochondrial ATP production and cellular energy regulation. Insufficient magnesium intake compromises mitochondrial health, impairing energy metabolism and increasing oxidative stress.
1. Magnesium’s Role in ATP Production
Magnesium is a cofactor for ATP synthase and stabilizes ATP molecules.
Nielsen (2010) explained that nearly all ATP in the cell exists as a magnesium-ATP complex, highlighting its indispensable role in energy transfer.
2. Mitochondrial Protection and Oxidative Stress
Magnesium reduces mitochondrial susceptibility to calcium overload and ROS-induced damage.
Barbagallo & Dominguez (2010) demonstrated that magnesium deficiency promotes oxidative stress and mitochondrial dysfunction.
3. Magnesium Deficiency and Chronic Disease
Low magnesium status is linked to increased risks of metabolic syndrome, type 2 diabetes, and cardiovascular disease, conditions associated with mitochondrial dysfunction.
Song et al. (2012) found that higher magnesium intake was inversely associated with type 2 diabetes risk.
Magnesium is a cornerstone nutrient for mitochondrial efficiency and protection. Ensuring sufficient magnesium intake is vital for energy production and overall metabolic health.
References:
Nielsen, F. H. (2010). Magnesium, inflammation, and obesity in chronic disease. Nutrients, 2(7), 583–616. DOI link
Barbagallo, M., & Dominguez, L. J. (2010). Magnesium and aging. Current Pharmaceutical Design, 16(7), 832–839. DOI link
Song, Y., et al. (2012). Magnesium intake, plasma magnesium levels, and the risk of type 2 diabetes: a meta-analysis of prospective cohort studies. Diabetes Care, 35(2), 374–382. DOI link
-
Omega-3 fatty acids, especially EPA and DHA, play a crucial role in mitochondrial membrane composition, reducing oxidative stress, and optimizing cellular energy production. These essential fats are linked to improved mitochondrial resilience, reduced inflammation, and enhanced metabolic function.
1. Omega-3s in Mitochondrial Membranes
DHA is incorporated into mitochondrial membranes, enhancing fluidity and membrane potential.
Stillwell & Wassall (2003) described how DHA improves mitochondrial membrane dynamics, supporting efficient electron transport and ATP synthesis.
2. Anti-Inflammatory Effects and Oxidative Protection
EPA and DHA reduce mitochondrial-derived ROS and activate anti-inflammatory pathways.
Calder (2015) highlighted that omega-3s reduce pro-inflammatory cytokines, lowering systemic and mitochondrial stress.
3. Omega-3s and Mitochondrial Biogenesis
Omega-3s activate PGC-1α and AMPK pathways, stimulating mitochondrial biogenesis and improving fatty acid oxidation.
Lombardo et al. (2017) reported that omega-3 supplementation enhances mitochondrial content and function in skeletal muscle.
Omega-3 fatty acids offer multifaceted support for mitochondrial structure and function. Their anti-inflammatory and energy-boosting properties make them essential for metabolic optimization and cellular vitality.
References:
Stillwell, W., & Wassall, S. R. (2003). Docosahexaenoic acid: membrane properties of a unique fatty acid. Chemistry and Physics of Lipids, 126(1), 1–27. DOI link
Calder, P. C. (2015). Marine omega-3 fatty acids and inflammatory processes: Effects, mechanisms, and clinical relevance. Biochimica et Biophysica Acta (BBA) - Molecular and Cell Biology of Lipids, 1851(4), 469–484. DOI link
Lombardo, Y. B., et al. (2017). Dietary fish oil modulates mitochondrial bioenergetics and fatty acid oxidation in skeletal muscle. Nutrition Research, 42, 25–33. DOI link