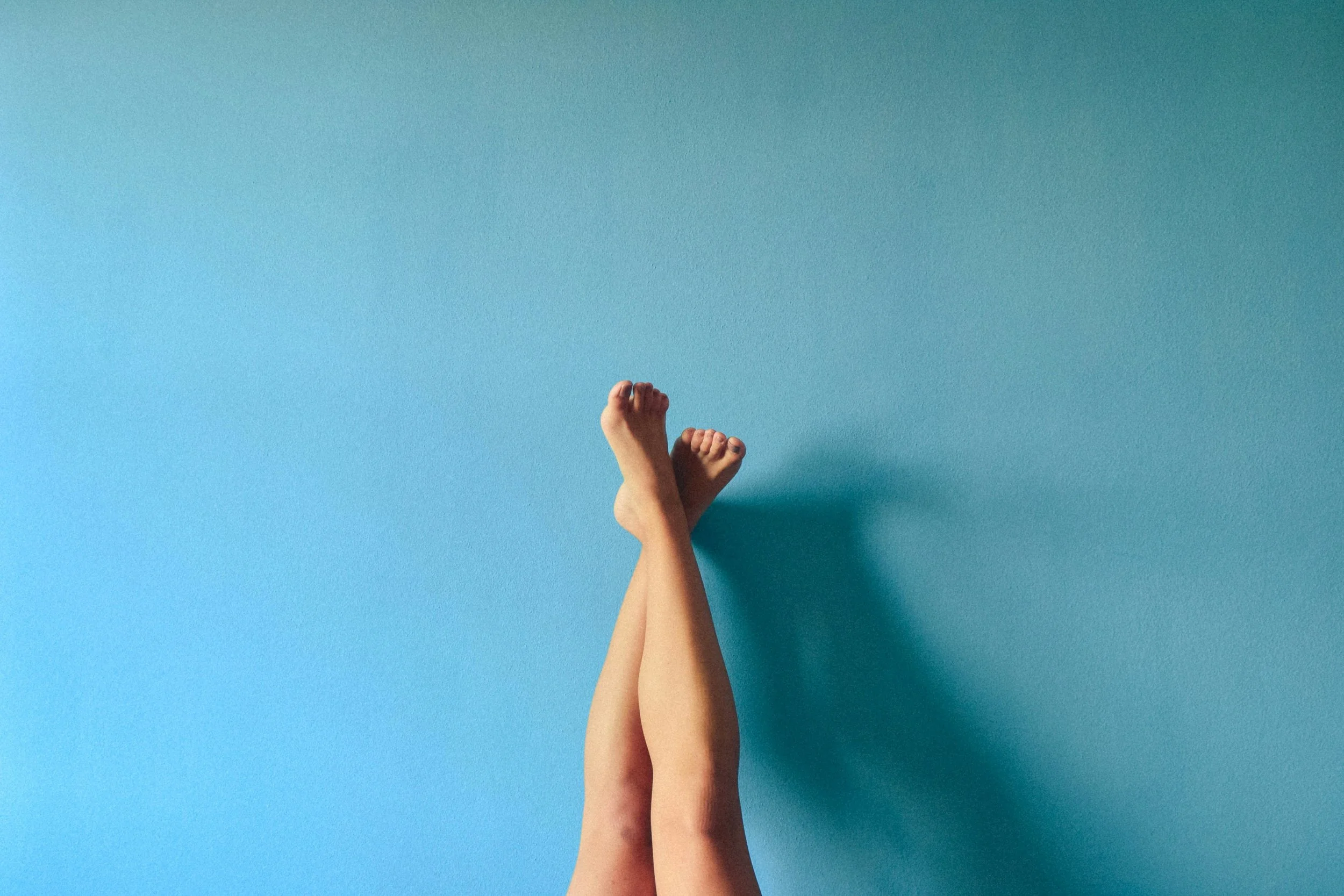
Metabolic therapeutics
Metabolic therapeutics offer a powerful, systems-based approach to healing by targeting the body’s core energy pathways. These strategies harness the science of metabolic flexibility—using fuel shifts, nutrient timing, and cellular stressors to recalibrate physiology at the mitochondrial level. By leveraging the body’s natural adaptive responses, metabolic therapeutics can reverse insulin resistance, lower inflammation, and enhance cellular resilience. In this section, we explore evidence-based interventions such as ketogenic therapies, fasting protocols, glucose and insulin modulation, and mitochondrial support. These articles dive into the therapeutic applications of metabolic manipulation—not just for managing chronic conditions, but for restoring metabolic integrity, cognitive clarity, and long-term vitality.

-
Metabolic flexibility refers to the body’s ability to switch between burning carbohydrates and fats for fuel depending on nutrient availability and energy demands. In a world of constant food access and chronic metabolic disease, restoring this flexibility is vital for metabolic health, performance, and longevity.
1. What is Metabolic Flexibility?
Metabolic flexibility allows cells to adapt fuel utilization between glucose and fatty acids based on the fed or fasted state.
Impaired metabolic flexibility, or “metabolic inflexibility,” is a hallmark of obesity, type 2 diabetes, and insulin resistance.
2. Mitochondria: The Metabolic Switchboard
Mitochondria are key regulators of metabolic flexibility.
Kelley et al. (2002) reported that insulin-resistant individuals exhibit mitochondrial dysfunction, leading to reduced fatty acid oxidation.
3. Insulin Resistance and Substrate Rigidity
In insulin-resistant states, cells rely excessively on glucose, impairing fat oxidation and contributing to lipid accumulation.
Improving insulin sensitivity through diet and exercise restores the balance between glucose and fat utilization.
4. Strategies to Enhance Metabolic Flexibility
Low-carbohydrate diets and ketogenic diets can retrain mitochondria to upregulate fat oxidation.
Intermittent fasting improves insulin sensitivity and stimulates ketone production, enhancing fat metabolism.
Aerobic and resistance training boost mitochondrial biogenesis and metabolic efficiency.
Metabolic flexibility is foundational to preventing and reversing metabolic dysfunction. By restoring fuel-switching capacity, clients can improve energy levels, resilience, and metabolic health.
References:
Kelley, D. E., He, J., Menshikova, E. V., & Ritov, V. B. (2002). Dysfunction of mitochondria in human skeletal muscle in type 2 diabetes. Diabetes, 51(10), 2944–2950. DOI link
Goodpaster, B. H., & Sparks, L. M. (2017). Metabolic flexibility in health and disease. Cell Metabolism, 25(5), 1027–1036. DOI link
Storlien, L., et al. (2004). Metabolic flexibility. Proceedings of the Nutrition Society, 63(2), 363–368. DOI link
-
Fasting is not just a strategy for caloric control; it triggers profound cellular processes, notably autophagy—the body's self-cleaning system. This process is pivotal for mitochondrial repair, immune rejuvenation, and metabolic resilience.
1. What is Autophagy?
Autophagy is a cellular recycling program where damaged proteins, organelles, and debris are broken down and reused.
The process is essential for maintaining mitochondrial health, preventing oxidative damage, and supporting longevity.
2. Fasting as a Catalyst for Autophagy
Nutrient deprivation, especially carbohydrate restriction, activates autophagy via inhibition of the mTOR pathway.
Mizushima & Komatsu (2011) described how fasting enhances autophagic flux in various tissues, promoting cellular renewal.
3. Metabolic and Immune Benefits
Autophagy enhances mitochondrial turnover (mitophagy), improving metabolic efficiency and reducing reactive oxygen species.
It also modulates immune responses by clearing dysfunctional immune cells and supporting anti-inflammatory cytokine profiles.
4. Clinical Implications
Fasting protocols (e.g., intermittent fasting, time-restricted eating) have shown benefits in metabolic syndrome, neurodegenerative diseases, and aging.
Madeo et al. (2019) highlighted fasting’s role in promoting healthy aging and reducing risk markers for diseases like Alzheimer’s.
Fasting-induced autophagy is a cornerstone of cellular rejuvenation and metabolic balance. Empower clients with sustainable fasting protocols to unlock natural self-repair mechanisms.
References:
Mizushima, N., & Komatsu, M. (2011). Autophagy: renovation of cells and tissues. Cell, 147(4), 728–741. DOI link
Madeo, F., et al. (2019). Caloric restriction mimetics against age-associated disease: targets, mechanisms, and therapeutic potential. Cell Metabolism, 29(3), 592–610. DOI link
Mattson, M. P., et al. (2017). Impact of intermittent fasting on health and disease processes. Ageing Research Reviews, 39, 46–58. DOI link
-
The ketogenic diet (KD) does more than shift macronutrient intake; it fundamentally alters mitochondrial function. By promoting ketone production and fat oxidation, KD enhances mitochondrial efficiency, reduces oxidative stress, and supports anti-inflammatory pathways, offering therapeutic potential for metabolic and neurological health.
1. Ketogenic Diets and Mitochondrial Bioenergetics
Ketosis increases mitochondrial respiration and ATP production while reducing ROS generation.
Bough et al. (2006) demonstrated that ketogenic diets improve mitochondrial biogenesis and membrane potential in animal models.
2. β-Hydroxybutyrate (BHB) as a Mitochondrial Modulator
BHB, the primary ketone body, inhibits histone deacetylases (HDACs), upregulating antioxidant genes.
Shimazu et al. (2013) revealed that BHB enhances oxidative stress defenses via FOXO3a and MnSOD activation.
3. Anti-Inflammatory and Neuroprotective Effects
Ketogenic diets suppress NLRP3 inflammasome activation, reducing systemic inflammation.
Youm et al. (2015) found BHB inhibits inflammatory responses, providing neuroprotective benefits.
4. Clinical Implications
KD is used therapeutically in epilepsy, metabolic syndrome, type 2 diabetes, and neurodegenerative diseases.
Enhanced mitochondrial health under KD supports improved insulin sensitivity and energy metabolism.
Ketogenic diets are a potent metabolic therapy, promoting mitochondrial resilience, reducing inflammation, and restoring metabolic balance. Coaches can leverage KD to help clients achieve cellular and systemic vitality.
References:
Bough, K. J., et al. (2006). Mitochondrial biogenesis in the anticonvulsant mechanism of the ketogenic diet. Annals of Neurology, 60(2), 223–235. DOI link
Shimazu, T., et al. (2013). Suppression of oxidative stress by β-hydroxybutyrate, an endogenous histone deacetylase inhibitor. Science, 339(6116), 211–214. DOI link
Youm, Y. H., et al. (2015). The ketone metabolite β-hydroxybutyrate blocks NLRP3 inflammasome–mediated inflammatory disease. Nature Medicine, 21(3), 263–269. DOI link
-
Carbohydrate restriction plays a critical role in managing type 2 diabetes (T2DM). Recent evidence demonstrates a dose-dependent relationship between the degree of carb restriction and improvements in glycemic control, insulin resistance, and weight loss.
1. The Carbohydrate-Glycemic Axis
Carbohydrate intake directly influences postprandial glucose and insulin levels.
Restricting carbohydrate intake reduces glycemic variability and improves insulin sensitivity.
Jayedi et al. (2022) found that greater carbohydrate restriction yielded larger reductions in HbA1c and fasting glucose levels.
2. Low to Very Low Carbohydrate Ranges
Moderate Carb Restriction (100–130g/day): Supports weight loss and glycemic improvements.
Low Carb (50–100g/day): Enhances insulin sensitivity, reduces triglycerides.
Very Low Carb/Ketogenic (<50g/day): Increases ketone production, optimizes glucose control.
3. The Meta-Analysis Evidence
In a dose-response meta-analysis, more severe carbohydrate restriction correlated with improved metabolic markers.
Goldenberg et al. (2021) reported that low-carb diets significantly outperformed low-fat diets for glycemic control in T2DM.
Carbohydrate restriction is a powerful lever in type 2 diabetes management, and the dose matters. Individualizing carbohydrate intake can yield significant clinical benefits.
References:
Jayedi, A., et al. (2022). Dose-dependent effect of carbohydrate restriction for type 2 diabetes management: A systematic review and dose-response meta-analysis. The American Journal of Clinical Nutrition, 116(1), 247–261. DOI link
Goldenberg, J. Z., et al. (2021). Efficacy and safety of low and very low carbohydrate diets for type 2 diabetes remission: Systematic review and meta-analysis. BMJ, 372, m4743. DOI link
Feinman, R. D., et al. (2015). Dietary carbohydrate restriction as the first approach in diabetes management. Nutrition, 31(1), 1–13. DOI link
-
Metabolic syndrome—characterized by insulin resistance, dyslipidemia, hypertension, and central obesity—has emerged as a major contributor to chronic disease risk. Intermittent fasting (IF) offers a practical intervention, improving metabolic flexibility, reducing inflammation, and reversing key biomarkers.
1. Defining Intermittent Fasting
IF encompasses dietary patterns such as time-restricted eating (TRE), alternate-day fasting (ADF), and 5:2 protocols.
These approaches cycle periods of eating and fasting to trigger metabolic and cellular adaptations.
2. Metabolic Benefits of IF
IF improves insulin sensitivity and lowers fasting glucose levels.
Mattson et al. (2017) showed IF activates autophagy, reduces oxidative stress, and improves mitochondrial function.
Harris et al. (2018) reported IF regimens are as effective as continuous calorie restriction for weight loss and cardiometabolic improvements.
3. Lipid and Inflammatory Markers
IF reduces triglycerides, LDL cholesterol, and inflammatory cytokines such as CRP.
Tinsley et al. (2019) found that TRE combined with resistance training improved body composition without compromising strength.
Intermittent fasting provides a highly adaptable therapeutic tool to address metabolic syndrome, balancing biological rhythms and metabolic processes.
References:
Mattson, M. P., et al. (2017). Impact of intermittent fasting on health and disease processes. Ageing Research Reviews, 39, 46–58. DOI link
Harris, L., et al. (2018). Intermittent fasting interventions for treatment of overweight and obesity in adults: A systematic review and meta-analysis. JBI Database of Systematic Reviews and Implementation Reports, 16(2), 507–547. DOI link
Tinsley, G. M., et al. (2019). Time-restricted feeding plus resistance training in active females: a randomized trial. The American Journal of Clinical Nutrition, 110(3), 628–640. DOI link
-
Our biological clock governs more than sleep; it orchestrates metabolic processes, including glucose regulation. Circadian misalignment—caused by late-night eating, shift work, or erratic schedules—can impair insulin sensitivity, elevate blood sugar levels, and increase chronic disease risk.
1. The Circadian Clock and Metabolism
The suprachiasmatic nucleus (SCN) regulates peripheral clocks in organs like the liver, pancreas, and adipose tissue.
Disruptions to circadian rhythms impair glucose uptake and insulin signaling.
2. Early Time-Restricted Feeding (eTRF) Insights
Jamshed et al. (2019) found eTRF (eating within a 6-hour window ending mid-afternoon) significantly reduced 24-hour glucose levels and improved insulin sensitivity.
Early feeding aligns nutrient intake with peak insulin sensitivity and mitochondrial function.
3. Chrono-Nutrition Studies
Scheer et al. (2013) demonstrated that hunger and glucose dysregulation naturally peak in the evening due to circadian patterns.
Consuming high-carbohydrate meals at night worsens glycemic responses and increases the risk of weight gain.
Synchronizing eating patterns with circadian biology enhances glucose regulation and supports long-term metabolic health.
References:
Jamshed, H., et al. (2019). Early time-restricted feeding improves 24-hour glucose levels and affects markers of the circadian clock, aging, and autophagy in humans. Cell Metabolism, 30(1), 92–105.e5. DOI link
Scheer, F. A. J. L., et al. (2013). The internal circadian clock increases hunger and appetite in the evening independent of food intake and other behaviors. Obesity, 21(3), 421–423. DOI link
Morris, C. J., et al. (2015). Circadian system, sleep and endocrinology. Endocrinology and Metabolism Clinics, 44(3), 435–454. DOI link
-
Autophagy is the body’s natural self-cleaning process, crucial for cellular renewal and resilience. Fasting, particularly intermittent fasting (IF) and prolonged fasts, stimulates autophagy, enhancing longevity, immune function, and protection against chronic disease.
1. The Cellular Cleanup Crew
Autophagy clears damaged organelles, misfolded proteins, and cellular debris.
Levine & Kroemer (2019) highlighted autophagy’s role in preventing neurodegeneration, cancer, and metabolic dysfunction.
2. Fasting as an Autophagy Trigger
Nutrient deprivation from fasting downregulates mTOR and activates AMPK, key pathways for autophagy initiation.
Madeo et al. (2019) showed fasting-induced autophagy improves mitochondrial quality and reduces inflammation.
3. Fasting and Longevity Pathways
Autophagy intersects with longevity pathways such as sirtuins, FOXO, and NAD+ metabolism.
Mattson et al. (2017) linked fasting-induced autophagy to improved cardiovascular and neurological health.
Fasting unlocks autophagy, empowering the body to heal and regenerate at the cellular level. This ancient biological mechanism offers a modern solution for resilience and vitality.
References:
Levine, B., & Kroemer, G. (2019). Biological functions of autophagy genes: A disease perspective. Cell, 176(1–2), 11–42. DOI link
Madeo, F., et al. (2019). Caloric restriction mimetics against age-associated disease: Targets, mechanisms, and therapeutic potential. Cell Metabolism, 29(3), 592–610. DOI link
Mattson, M. P., et al. (2017). Impact of intermittent fasting on health and disease processes. Ageing Research Reviews, 39, 46–58. DOI link
-
Mitochondria are the metabolic engines of our cells, converting nutrients into ATP and regulating oxidative stress. Metabolic therapies—such as ketogenic diets, fasting, and carbohydrate restriction—enhance mitochondrial function, reduce inflammation, and support metabolic resilience.
1. Mitochondria at the Core of Metabolic Health
Mitochondria govern energy production, redox balance, and apoptosis.
Mitochondrial dysfunction contributes to insulin resistance, obesity, and neurodegenerative diseases.
2. Ketogenic Diet and Mitochondrial Efficiency
Parker et al. (2018) found that beta-hydroxybutyrate (BHB) improves mitochondrial biogenesis and fatty acid oxidation in skeletal muscle.
Ketogenic diets increase mitochondrial coupling efficiency, reducing ROS production.
3. Fasting and Mitochondrial Renewal
Fasting induces mitophagy, the targeted recycling of damaged mitochondria.
Madeo et al. (2019) linked caloric restriction and fasting-mimicking diets to enhanced mitochondrial quality control.
4. Exercise and Mitochondrial Adaptation
Resistance and endurance training stimulate mitochondrial biogenesis via PGC-1α activation.
Combined with nutritional strategies, exercise amplifies mitochondrial density and oxidative capacity.
Strengthening mitochondrial function is foundational for metabolic resilience and cellular vitality. A targeted, integrative approach optimizes energy production and reduces oxidative stress.
References:
Parker, M. D., et al. (2018). β-hydroxybutyrate elicits favorable mitochondrial changes in skeletal muscle. Journal of Clinical Investigation Insight, 3(23), e122534. DOI link
Madeo, F., et al. (2019). Caloric restriction mimetics against age-associated disease: Targets, mechanisms, and therapeutic potential. Cell Metabolism, 29(3), 592–610. DOI link
Wallace, D. C. (2010). Mitochondrial dysfunction in disease and aging. Environmental and Molecular Mutagenesis, 51(5), 440–450. DOI link
-
Low-carbohydrate and ketogenic diets induce a metabolic shift from carbohydrate reliance to fat oxidation. This adaptation enhances metabolic flexibility, improves endurance capacity, and supports metabolic health.
1. The Metabolic Switch
In carbohydrate-restricted states, the body prioritizes lipolysis and ketogenesis.
Volek et al. (2016) found that keto-adapted ultra-endurance athletes exhibited significantly higher rates of fat oxidation, even at high intensities.
2. Energy Efficiency and Performance
Keto-adapted athletes rely on fat stores for energy, preserving glycogen and reducing dependency on exogenous carbohydrates.
Enhanced fat oxidation supports sustained energy during prolonged physical exertion.
3. Low-Carb Diets and Weight Management
Shai et al. (2008) demonstrated superior weight loss and improved lipid profiles on low-carbohydrate diets compared to low-fat diets.
Fat oxidation contributes to reductions in visceral fat and improvements in insulin sensitivity.
Low-carbohydrate diets foster metabolic efficiency, enhance fat oxidation, and promote metabolic health. This approach can be tailored for athletes, weight management, or chronic disease prevention.
References:
Volek, J. S., et al. (2016). Metabolic characteristics of keto-adapted ultra-endurance runners. Metabolism: Clinical and Experimental, 65(3), 100–110. DOI link
Shai, I., et al. (2008). Weight loss with a low-carbohydrate, Mediterranean, or low-fat diet. New England Journal of Medicine, 359(3), 229–241. DOI link
Phinney, S. D., et al. (1983). The human metabolic response to chronic ketosis without caloric restriction: preservation of submaximal exercise capability with reduced carbohydrate oxidation. Metabolism, 32(8), 769–776. DOI link
-
The carbohydrate-insulin model (CIM) challenges traditional energy balance theory by emphasizing hormonal regulation, particularly insulin, as a driver of obesity and metabolic dysfunction. This model highlights how macronutrient composition, not just caloric intake, influences fat storage and energy utilization.
1. The Carbohydrate-Insulin Model Explained
Carbohydrate consumption stimulates insulin secretion, promoting fat storage and inhibiting fat mobilization.
High-insulin states, common in high-glycemic diets, reduce metabolic flexibility and contribute to energy trapping within adipose tissue.
2. Evidence Supporting CIM
Ludwig et al. (2021) proposed that refined carbohydrates dysregulate insulin responses, promoting fat accumulation independent of calorie surplus.
Ebbeling et al. (2012) found that very-low-carbohydrate diets preserved higher total energy expenditure compared to low-fat diets during weight-loss maintenance.
3. Competing Paradigms: CIM vs. Energy Balance Model
The traditional energy balance model (EBM) emphasizes caloric excess.
CIM accounts for hormonal responses and metabolic adaptations beyond calorie counting.
The carbohydrate-insulin model offers a nuanced understanding of obesity, linking hormonal responses to diet quality and metabolic health. Integrating CIM principles can empower clients to unlock sustainable fat loss and metabolic vitality.
References:
Ludwig, D. S., et al. (2021). The carbohydrate-insulin model: a physiological perspective on the obesity pandemic. European Journal of Clinical Nutrition, 75(4), 556–565. DOI link
Ebbeling, C. B., et al. (2012). Effects of dietary composition on energy expenditure during weight-loss maintenance. JAMA, 307(24), 2627–2634. DOI link
Hall, K. D., et al. (2019). Ultra-processed diets cause excess calorie intake and weight gain: An inpatient randomized controlled trial of ad libitum food intake. Cell Metabolism, 30(1), 67–77.e3. DOI link